By Uno Mutlu, Director of Business Strategy & Principal Scientist at New England Research Inc.
Emerging subsurface engineering applications—from nuclear waste disposal for small modular reactors to geologic hydrogen production, CO₂ sequestration, thermal energy storage, superhot-supercritical geothermal systems, and critical mineral extraction—are poised to redefine our energy landscape. Each technology promises low-carbon, affordable, and secure energy supplies. Yet, despite their diverse aims, all share a need to operate safely and efficiently within the Earth’s complex subsurface environment. Achieving these objectives demands precise, predictive characterization & modeling of highly coupled thermo-hydro-mechanical-chemical (THMC) processes. Historically, numerical simulation of these processes has been hampered by several hurdles: lack of data, the sheer computational cost of modeling subsurface systems and sensitivity analysis, the deep specialized expertise required to build and interpret advanced models, and the incomplete coupling of thermo-hydro-mechanical-chemical physics in many existing tools. These barriers have limited the broader adoption of digital twins—virtual, predictive models capable of capturing the intricacies of subsurface behavior. Recent technological breakthroughs, however, are rapidly changing this landscape. Integration between New England Research’s (NER) testing capabilities with modern software solutions, such as Irazu from Geomechanica are closing the gap between scientific rigor and practical application.
By leveraging GPU acceleration and sophisticated numerical algorithms, these platforms can tackle large-scale, high-resolution THMC simulations with unprecedented speed and accuracy within an intuitive UI. As a result, they enable a wider range of stakeholders—beyond PhD-level experts—to engage with critical subsurface design challenges. This democratization of advanced modeling fosters communication between teams and better-informed decisions: helping operators balance operational efficiency with minimized environmental risk.
For example, applications like stimulated geologic hydrogen hinges on understanding subsurface deformation and progressive cracking behavior under HTHP conditions. Intuitive yet advanced THM modeling capabilities lay a solid foundation to decode this behavior while providing for safe, predictive, and cost-effective project designs. Through proof-of-concept numerical models, we can further our understanding of micro-cracking driven by autonomous THMC processes and have the opportunity to engineer natural hydrogen producing systems that are commercially viable.
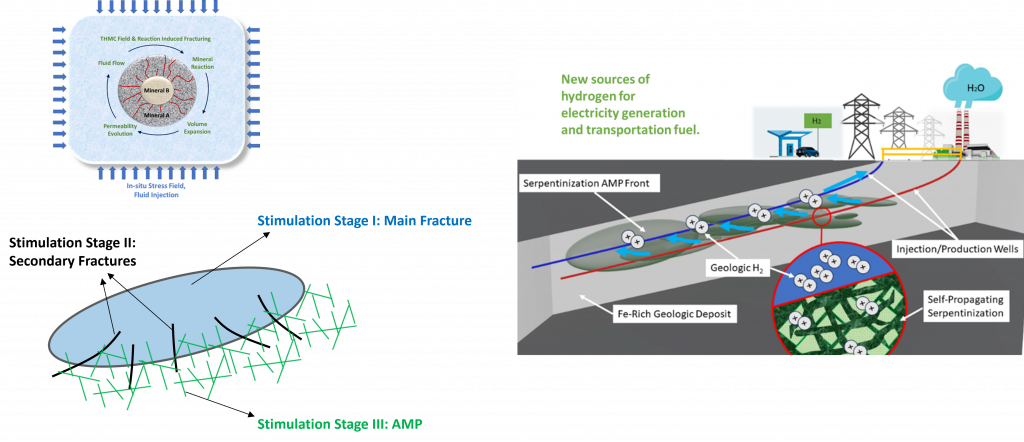
One way hydrogen is produced is through serpentinization where olivine-pyroxene rich mafic and ultramafic rocks interact with water under specific temperature and pressure conditions. This reaction is accompanied by significant volumetric expansion promoting the development of micro-crack networks. These micro-cracks can enhance permeability, enabling continuous water flow and subsequent mineralization, creating a self-sustaining feedback loop. This iterative process can progressively transform the rock’s permeability, making it more conducive to hydrogen generation.
To better understand this mechanism and engineer it for commercial viability, a digital twin model was created in Irazu whereby a scanning electronic microscope image was directly inputted to capture all key geometric features of the rock, including the serpentinized vein network and the sub-grain geometries. Using a TM-coupled model, volumetric expansion of the serpentinized material is approximated through heat conduction and thermal expansion as a proxy for the progression of the hydration front and reaction induced volumetric strain increases within the veins. As the process progresses in time, local stress concentrations from the expansion reaction leads to the gradual widening of the veins, ultimately enhancing the micro-crack network. The sequence begins predominantly with micro-cracking along grain boundaries and transitions to micro-cracking within the grains. In the late phases of the process, there exists a distribution of grains saturated with micro-cracks and those that remain under-saturated. Hence, there may be an opportunity to artificially stimulate these under-developed areas and further enhance hydrogen production.
By developing and refining advanced digital twins for hydrogen production, we not only enable safer and more efficient resource extraction but also bolster a critical and rapidly expanding market, positioning hydrogen as a cornerstone of sustainable economic growth in the global energy transition. As we enter this exciting new chapter in subsurface engineering, predictive digital twins—integrated by HTHP data and supported by innovative modeling tools like Irazu—will be the key to unlocking the full potential of these transformative technologies.
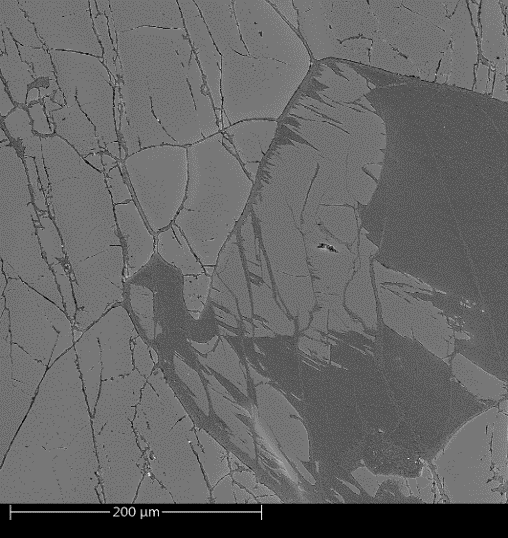
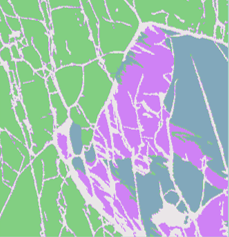
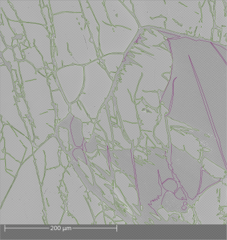
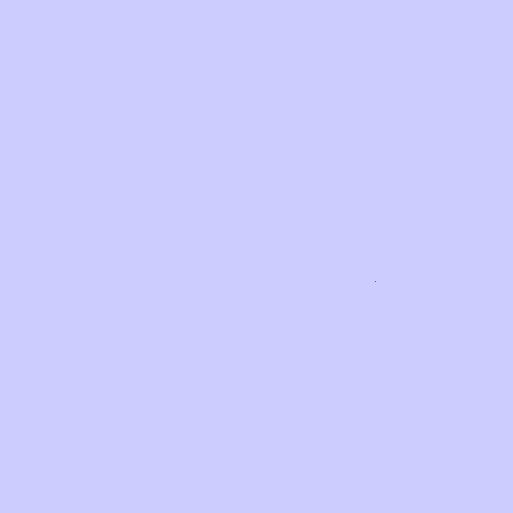
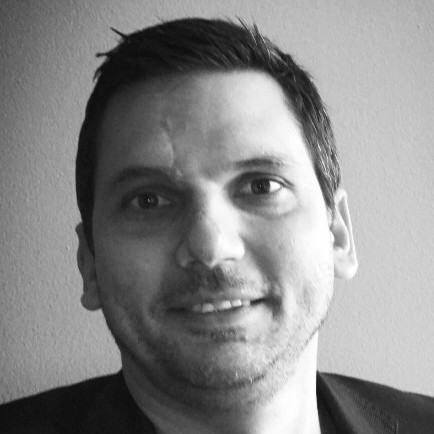
Uno Mutlu is the Director of Business Strategy & Principal Scientist at New England Research Inc. He is a proactive, globally experienced leader holding a PhD in engineering, with extensive industry experience across clean energy, oil & gas, environmental sciences, computational/experimental mechanics, infrastructure and decarbonization, including specialized areas like CO2 storage, hydrogen, and geothermal. Uno possesses a proven track record of building large technical teams from the ground up and driving business strategy, evidenced by past leadership roles such as Director of Strategy at Corva, COO at Rockfield Americas, and R&D Manager at Weatherford. His background is further supported by over 50 publications/patent applications and research experience at ExxonMobil and Stanford, showcasing expertise in developing innovative solutions and integrating advanced measurements with modeling for data-driven decision-making in energy and related sectors.